In the design project to replace the old Fulton Road arch bridge in Cleveland, OH, Michael Baker Intl engineer Daniel Baxter and his team has designed a 1,568-foot-long replacement structure for the original arch bridge, which retains the original design of six 210-foot-long concrete deck arch spans. For the replacement bridge structure, a precast, post-tensioned concrete arch bridge design was selected. You may be asking yourself, why post-tensioning the arch bridge? Arches usually provide sufficient stabilities to structures and it is not usual for bridge designers to post-tension such structures. In this tip, Daniel Baxter talks about why his team has decided to post-tension the arch bridge and how they have utilized construction stage analysis to design the post-tension process.
Left: The original Fulton Street Bridge structure (now demolished). Right: Aerial view of the new Fulton Street Bridge.
The image blow shows the arch layout of one of the six arch spans, where there are four arch ribs throughout the lateral span. Four spandrel columns also sit on top of the arch ribs supporting the superstructure.
In this case, the combination of the arch sitting on the piers which have a fair amount of flexibility and the spandrel columns being widely spaced (42 ft) introduces concentrated load on the 4 locations on the arch. These factors also create more bending on the arch than the traditional arch. Using bending moment diagram of the arch under dead load and live load, shown below, we can understand why we are seeing such large bending moment during the design process. We started with the very basic arch on rigid support (red), then we compare the bending moment curve of the arch with actual support which have rigid support (blue) and with flexibilities (green). Eventually, we obtained the bending moment curve (gold) of the bridge structure with influence of the piers with flexibility of their foundations, and also adding in the effect of construction sequence analysis produced in midas Civil software.
When we look at the stresses in the arch, shown below, we can observe few regions where the stress exceeds the AASHTO limits, particularly near the end segments where the end segments meets the pier bases. Depending on the live load, the stress level at the intermediate spandrel columns also exceed the stress limits. This design has fewer spandrel columns than traditional arches, therefore, more stress is introduced and we have chosen post-tension in order to meet the stress limits requirement.
However, more challenges came up with the post-tensioning design. These arches have fixes stands to them, and if engineers try to use eccentric post tensioning to counteract bending moments, those fixed stands will experience significant restraints. This would result in secondary moment that will cancel out any beneficial primary prestress moment obtained from eccentric post-tensioning. Therefore, construction stage analysis is used that introduces eccentric post-tensioning was introduces during the structural configuration that does not have the full rigidity of the completed arch. Because it does not have the full rigidity, the segment is able to deform due to the eccentric pre-stress force, and thus introducing beneficial primary pre-stress moments to counteract bending moment.
Therefore, as shown in the figure below, after the end segments are erected with temporary towers, two 19 strand eccentric post-tensioning tendons are stressed on two end segments. As stated above, the eccentricity imparts beneficial primary pre-stress moment into the arch to counteract with the bending moment coming in from the superstructure. At the time the prestress force is introduced, the arch is not closed, giving flexibility to the system of the arch being supported by the temporary arch towers.
The next step, as shown in the figure below, is tow pre-stress the crown segment using two 19 strand post-tensioning tendons. The post-tensioning happens before the segment is set on the tower so that it could take place on the ground without running into any clearance issues with end segments.
Then, as shown in the figure below, crown segments are erected, the closure pours were placed between the segments, and the duct connections were made for the post-tensioning ducts.
Finally, four 15 strand post-tensioning tendons are placed through arch span for continuity and with symmetric configuration (one on each corner). They exerts pulling force on the arch and makes the arch rib compress against itself to add on more axial compression to the system. They also do not add on any prestress moment due to lack of any net eccentricity.
Now when we look at the service level stress throughout the arch span after the post-tensioning, with the effects of dead load and live load included, we observe more axial compression, shown in the figure below. We are also further away from exceeding the maximum allowable tensile stress because the post-tensioning of the arch span has created beneficial compression that is able to resist bending.
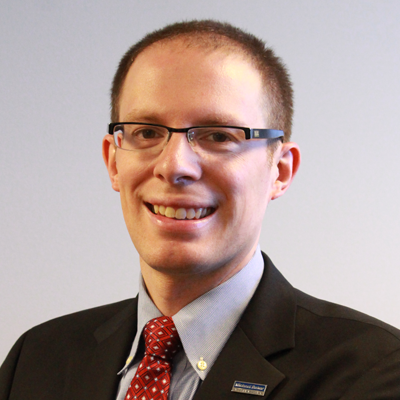
Daniel Baxter is a bridge department manager in Michael Baker's Minneapolis office, where he also serves as a project manager and senior bridge engineer. He has been involved in both the rehabilitation, design, and load rating of many complex bridges such as the Third Avenue Bridge, Winona Bridge, High Bridge, Franklin Avenue Bridge, Lafayette Bridge and Hastings Bridge, all over the Mississippi River. He also recently led the analysis of 18 existing pier caps with sectional and strut-and-tie methods and helped develop procedures for the design of 38 new pier caps using the strut-and-tie method. He also serves as an NHI-certified instructor for multiple NHI bridge design and load rating training courses, including Strut-and-Tie Modeling for Concrete Structures. He is highly experienced in complex finite element analysis, has given eight presentations at the International Bridge Conference, and has published several papers in peer-reviewed journals. He graduated with a B.A. in Physics from Carleton College in 2001 and received a B.S. in Civil Engineering and an M.S. in structural engineering in December 2003 from Washington University in St. Louis. He is a licensed professional engineer in Minnesota and a licensed structural engineer in the state of Illinois.